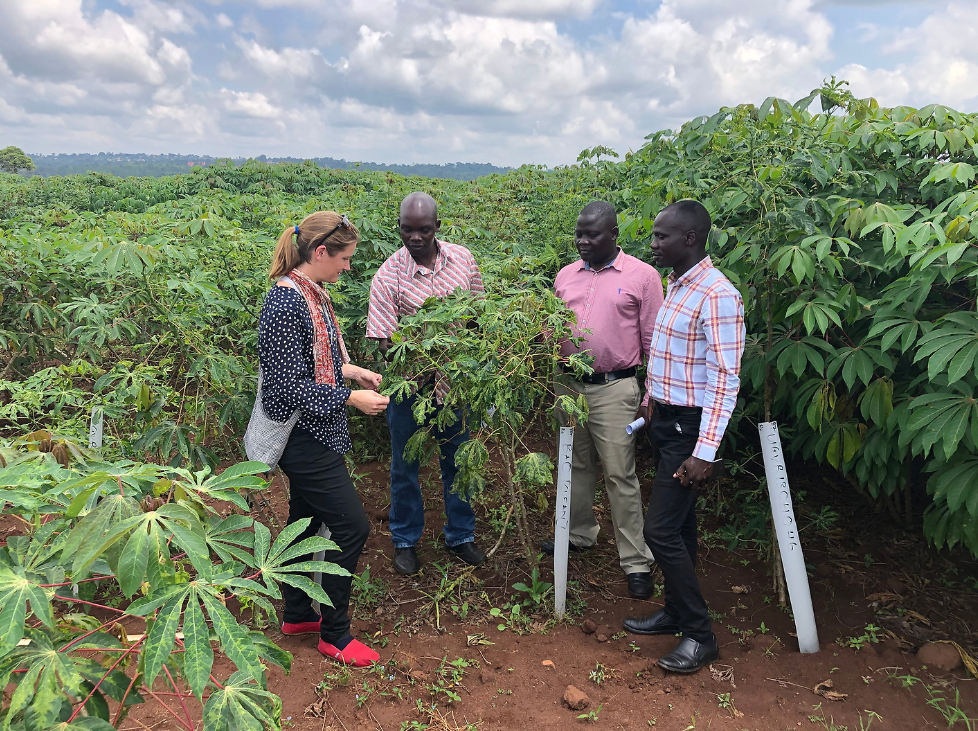
Geminivirus Resistance
Unveiling the Latest Projects in the Bart Lab
Cassava is an important crop for small, medium, and large-scale farmers. It is a hardy plant that can be grown without irrigation, fertilizer or pesticides and is highly productive even when grown on marginal land. As such, it is likely to become an even more important crop in the face of global climate change. Cassava Mosaic Disease (CMD) is a devastating disease of cassava across the African continent and has recently spread to Asia. Some varieties of cassava have natural genetic resistance to CMD and the gene responsible for this resistance was recently identified. However, it is not yet understood how the resistance works. With hundreds of millions of people depending on this one source of resistance to a devastating pathogen, a deeper understanding of the mechanism behind the resistance is desired. This research will reveal the molecular mechanisms of resistance and test whether this type of resistance can protect diverse and important crops from other virus pathogens. If successful, this research will lead to sustainable and effective disease control strategies for many important crops such as tomato and cotton. Throughout the research, training will be provided for undergraduate and graduate students and the importance of this research will be shared with society through a variety of public lectures, outreach events and YouTube videos explaining the importance of this specific project targeted at non-scientist audiences.
CMD is caused by species of DNA geminiviruses. The CMD resistance was tracked to specific amino acid changes within the DNA polymerase delta subunit 1 (POLD1) protein. Viruses are fantastically effective at overcoming host resistance mechanisms and yet this resistance trait has been stable for decades. Further, current data support the hypothesis that several resistant cultivars of cassava are periclinal chimeras with the resistance allele present in only specific cell layers. Why this resistance is so stable and how it can function in the context of a periclinal chimera, is not yet clear. While some of the identified POLD1 mutations are novel, others have been observed in yeast and result in decreased DNA replication fidelity, pointing towards a possible functional mechanism. Transient assays will be used to test this and other candidate mechanistic hypotheses. These include characterizing viral replication rates and fidelity of the different POLD1 alleles, interaction with candidate viral and host co-factors and protein crystallography. Beyond cassava, this research may yield new resistance strategies for other important crops. A resistant POLD1 allele will be transformed into the model system Arabidopsis, to directly test if this resistance mechanism is effective in a distinct pathosystem. In addition, exploration of publicly available genomic data suggests that similar alleles exist in germplasm collections from tomato, cotton, and several other important crops. The relevant germplasm has been obtained and will be challenged with the respective viral pathogens. This project is generously supported by the National Science Foundation (NSF: 2323058) and the USDA-NIFA through their joint Plant Biotic Interactions program (NSF: 2023-67013-41103).
Plant Microbiomes
Sustainable agricultural practices are necessary to mitigate climate change and produce more food, fiber and renewable fuels. An untapped frontier in agriculture is to promote beneficial interactions with microbes as a sustainable mechanism of providing nutrients to crops. ‘Microbial’ products are already available to farmers; however, in practice, these products display highly variable results and current data suggest that this is tightly linked to the ability of the beneficial microbes to colonize and persist with their plant hosts. Plant root exudates are a major factor that determine the colonization and persistence phenotypes of exogenously supplied microbes; however, our knowledge of how plant exudates affect colonization and persistence is too limited to be translated into improved performance of microbial products. In this project, we combine field level, computational observations with lab based, reductionist experiments. Together, these approaches will yield new synthetic communities of bacteria with plant growth promoting abilities and fundamental knowledge of how plant root exudates affect the colonization and persistence of the synthetic communities.
In this project, multivariate datasets on root and shoot phenotypes from a field trial of 16 diverse maize lines are being leveraged with 323 candidate beneficial bacteria identified in the same study to computationally predict co-occurring or exclusionary (never co-occurring) microbial community members that lead to enhanced growth and plant productivity. Microbes within the communities are being isolated and characterized by genome sequencing and high throughput bioassays. Synthetic communities (SynComs) will be assembled based on results from the co-occurrence and biochemical analyses. In addition, we are developing new methods of directly detecting nutrient exchange between plant roots and the microbial community, for example, using isotopic labeling and metabolite profiling. This work is part of the Danforth Center’s Subterranean Influences on Nitrogen and Carbon (SINC) Center of Excellence, a collaboration with Doug Allen’s lab and is generously supported by the USDA-NIFA Agricultural Microbiomes program (NSF: 2023-67017-39419).
Targeted epigenome editing for crop disease resistance
Pathogens rely on expression of host susceptibility (S) genes to promote infection and disease. As DNA methylation is an epigenetic modification that affects gene expression, blocking access to S genes through targeted methylation could increase disease resistance. In this project, we are using targeted methylation technologies, developed in Steve Jacobsen’s lab, to increase tolerance to important bacterial and viral diseases in the food security crop, cassava. In the first case, Xanthomonas phaseoli pv. manihotis, the causal agent of cassava bacterial blight (CBB), uses transcription activator-like 20 (TAL20) to induce expression of the S gene MeSWEET10a. We directed methylation to the TAL20 effector binding element within the MeSWEET10a promoter using a synthetic zinc-finger DNA binding domain fused to a component of the RNA-directed DNA methylation pathway. We demonstrated that this methylation prevents TAL20 binding, blocks transcriptional activation of MeSWEET10a in vivo and that these plants display decreased CBB symptoms while maintaining normal growth and development. In the second case, we are collaborating with Jim Carrington’s lab and focus on a class of RNA viruses that cause cassava brown streak disease (CBSD). Using similar DNA methylation targeting technologies, we have established de novo methylation at multiple target sites. This work is funded by the Bill and Melinda Gates Foundation (INV-002958).
With new funding from the National Science Foundation (NSF PGR: 2331437) and in collaboration with Bing Yang’s lab, we are currently working to deploy these tools in both rice and tomato, testing additional generations of the methylation targeting reagents developed by the Jacobsen lab and investigating heritability of novel epi-alleles.